Introduction
Animals around the world face extreme challenges in winter as temperatures drop significantly, especially in polar and high-altitude regions. The ability to survive in such harsh environments is vital for many species, and over time, animals have evolved an array of adaptations—both physiological and behavioral—to cope with these conditions. These adaptations are not only crucial for maintaining body temperature but also for ensuring survival during periods of food scarcity and limited daylight.
In the polar regions, temperatures often plummet to −40°C or lower during winter months, creating a difficult environment for many animals. For homeothermic animals—those that regulate and maintain a stable internal body temperature—these extreme cold conditions are particularly challenging. Maintaining internal heat in freezing temperatures requires significant energy and specialized physical traits, such as thick fur, blubber, or enhanced metabolic processes. The cold also brings other difficulties, such as food scarcity and lack of shelter, forcing many species to either migrate, hibernate, or develop other behaviors to endure.
Understanding how animals adapt to such cold environments provides insight into the remarkable resilience of life. These adaptations ensure the survival of various species, from mammals and birds to insects, and help them continue thriving despite the challenges of their surroundings.
How Winter Impacts Animal Physiology
Animals living in cold environments have developed several physiological mechanisms to minimize heat loss and survive extreme temperatures. One key strategy is through specialized vascular arrangements that allow counter-current heat exchange. In species such as Arctic dogs, reindeer, and sea birds, the legs and appendages—areas with less insulation—are maintained at much lower temperatures than the core body. This adaptation is achieved through anatomical structures where warm arterial blood flows close to cooled venous blood, allowing the arterial blood to be cooled before reaching extremities, thus minimizing heat loss. In some animals, like reindeer, the heat exchange is highly efficient in the long limbs, while in seals, the shorter flippers have a more intricate vascular arrangement, allowing parallel vessels for improved heat conservation.
In Arctic wolves and foxes, vascular plexuses in the paws help maintain foot temperatures close to 0°C, even in extremely cold conditions (−50°C). This mechanism involves sophisticated nerve and vascular control, as the peripheral nerves and blood vessels remain functional at temperatures approaching freezing. The adaptation also involves changes in the composition of tissues, such as bone marrow and hemoglobin, which help prevent freezing and facilitate oxygen delivery in cold environments.
Hibernation: A Vital Survival Strategy
Hibernation is a survival strategy that allows animals to endure harsh winters and food scarcity. Black and grizzly bears exhibit physiological changes similar to deep hibernators, such as reduced heart rate, metabolic rate, and body temperature. However, unlike bats and rodents, which lower their body temperature to nearly 0°C and periodically wake up, bears hibernate continuously for 3–7 months while maintaining a body temperature of 31–35°C.
During this period, black bears do not eat, drink, urinate, or defecate but still expend about 4,000 kcal per day. Despite their dormancy, they can react to disturbances, and females even give birth and nurse their cubs. Unlike other animals that break down muscle protein for energy, hibernating bears preserve lean body mass, losing weight only from fat stores. They also prevent nitrogen waste accumulation by reabsorbing urine through the bladder wall, avoiding harmful urea buildup that could disrupt hibernation.
A key adaptation in bears is their ability to prevent ketosis. While many fasting animals accumulate ketone bodies, bears show only slight increases, maintaining a balanced metabolism. This is achieved through efficient fat utilization, where glycerol from fat breakdown contributes to glucose production, supporting protein synthesis. Other hibernators, like hoary marmots, develop ketonemia, which may act as a trigger for periodic waking.
In conclusion, black and grizzly bears exhibit a highly specialized form of hibernation that conserves energy, maintains muscle mass, and prevents metabolic disorders. Their ability to recycle urea, efficiently use fat, and avoid ketosis allows them to survive months of dormancy without food or water, making them remarkable hibernators.
Additionally, Arctic mammals and birds have evolved nasal heat and water conservation systems to prevent excessive evaporative loss. In species like reindeer, specialized nasal structures increase surface area for heat exchange. As cold air is inhaled, it is heated and humidified before reaching the lungs, while exhaled air is cooled and dehumidified, conserving both heat and moisture. This system, present in both reindeer and seals, is critical for survival in extreme cold, with seals retaining up to 80% of the water and 65% of the heat from inspired air. These adaptations enable animals to maintain body temperature and conserve energy in the harshest environments.
Metabolic Regulation in Polar Bears
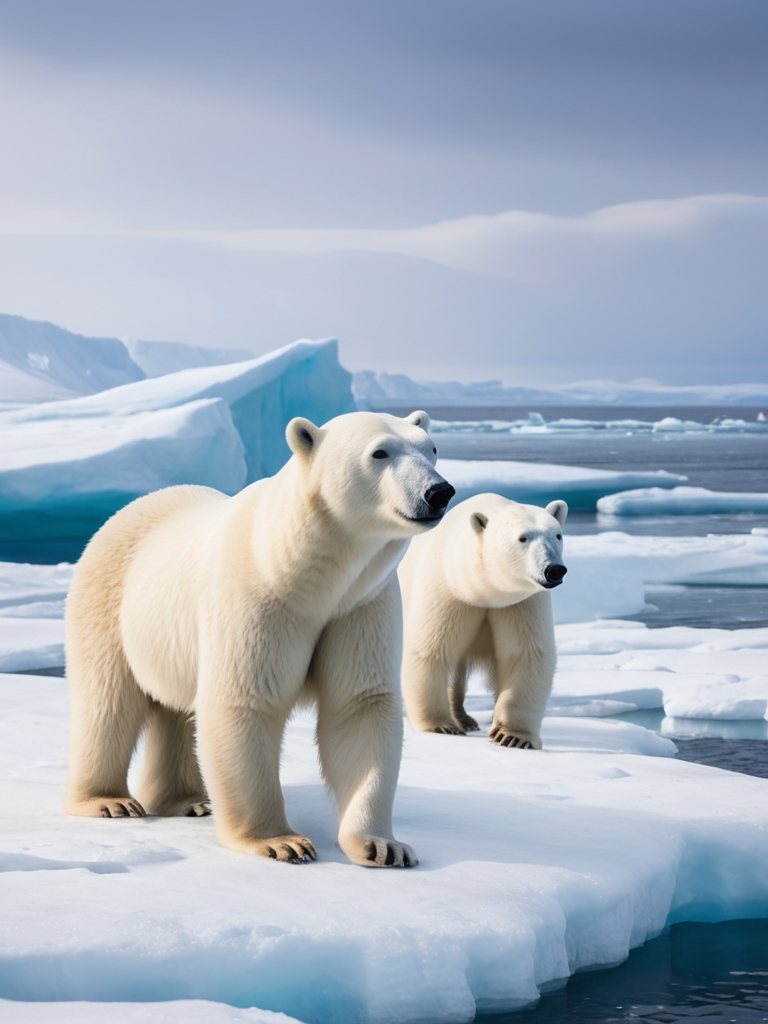
Polar bears exhibit unique metabolic adaptations that allow them to survive harsh winter conditions while maintaining their energy balance. During summer, wild polar bears in Churchill, Manitoba, show biochemical signs of hibernation despite remaining active and consuming little food. Unlike other hibernators, they do not enter deep torpor but instead rely on fat reserves, remaining lethargic and limiting their food intake. Observations suggest that their metabolic state in summer allows them to conserve energy without the need for frequent feeding or water intake.
In winter, polar bears display both activity and periods of denning. Pregnant females spend the longest time in dens, which suggests they may enter a deeper hibernation-like state. However, other polar bears remain active, engaging in intermittent feeding, primarily on seal blubber, which aligns with Stage II. Unlike traditional hibernators that rely on carbohydrate metabolism, polar bears maintain energy levels by consuming large amounts of fat while minimizing protein intake. This metabolic strategy prevents disruption of hibernation-like biochemical processes, as digesting protein would increase nitrogen waste and water loss.
The regulation of food intake in polar bears appears to be linked to fat reserves. When fat cell size decreases beyond a critical threshold, a biological signal may trigger increased fat consumption. This mechanism ensures energy balance during periods of limited food availability. Additionally, since fat metabolism produces water as a byproduct, polar bears can maintain hydration without consuming large amounts of snow or ice, reducing the energy costs associated with warming ingested water.
Unlike other animals that experience muscle loss during prolonged fasting, polar bears may preserve lean body mass by relying solely on fat metabolism, similar to hibernating black bears. This adaptation prevents the depletion of essential proteins and minimizes the need for frequent food intake. Although lactating and growing bears require protein for milk production and development, their protein intake likely serves growth rather than being broken down for energy.
Overall, the metabolic regulation in polar bears allows them to endure extreme Arctic conditions with minimal food and water intake while sustaining essential biological functions. Their ability to selectively metabolize fat, conserve protein, and maintain energy levels through intermittent feeding provides a survival advantage in one of the world’s harshest environments
Winter Adaptation in Aquatic Animals
Arctic and Tropical Fish
The key difference between Arctic and tropical fishes lies in their thermal adaptation. Arctic fish can survive in near-freezing waters but are unable to tolerate temperatures above 10-20°C. In contrast, tropical fish cannot survive in very cold temperatures, with some dying below 15-20°C and all perishing by 40°C. Additionally, tropical fish have significantly higher oxygen consumption rates—roughly four to five times greater than Arctic fish of the same weight. The metabolic response to temperature changes remains relatively constant, typically between 2 and 3.
Arctic and Tropical Crustaceans
Similar to fish, Arctic crustaceans are adapted to cold environments, remaining active even in freezing waters. Their metabolic rate is considerably lower than that of tropical crustaceans, which have rates five to six times higher. Some species, such as the freshwater phyllopod Lepidurus, show exceptionally high metabolic activity. Among tropical crustaceans, fast-moving species like the “sea roach” and fiddler crab exhibit relatively lower oxygen consumption. Despite these differences, both Arctic and tropical crustaceans maintain a stable metabolic response within their natural temperature ranges.
Arctic and Tropical Mollusks
Arctic mollusks, including slugs and amphibious snails, display unique metabolic adaptations. They exhibit a higher oxygen consumption rate below 30°C compared to a Venezuelan snail. When comparing Arctic and tropical species at 0°C and 20°C, Arctic mollusks tend to consume slightly more oxygen at lower temperatures, a trend that becomes more pronounced at 10°C. Their metabolic rate significantly decreases below 10°C, while tropical species maintain a relatively stable response. Arctic mollusks can survive freezing conditions (0°C) and tolerate temperatures up to 40°C, whereas tropical snails are sensitive to both extreme cold and high temperatures.
This study highlights the significant physiological adaptations that allow Arctic aquatic animals to thrive in cold environments, contrasting with the higher metabolic demands and limited cold tolerance of their tropical counterparts.
The Wood Frog (Rana sylvatica): Nature’s Frozen Survivor
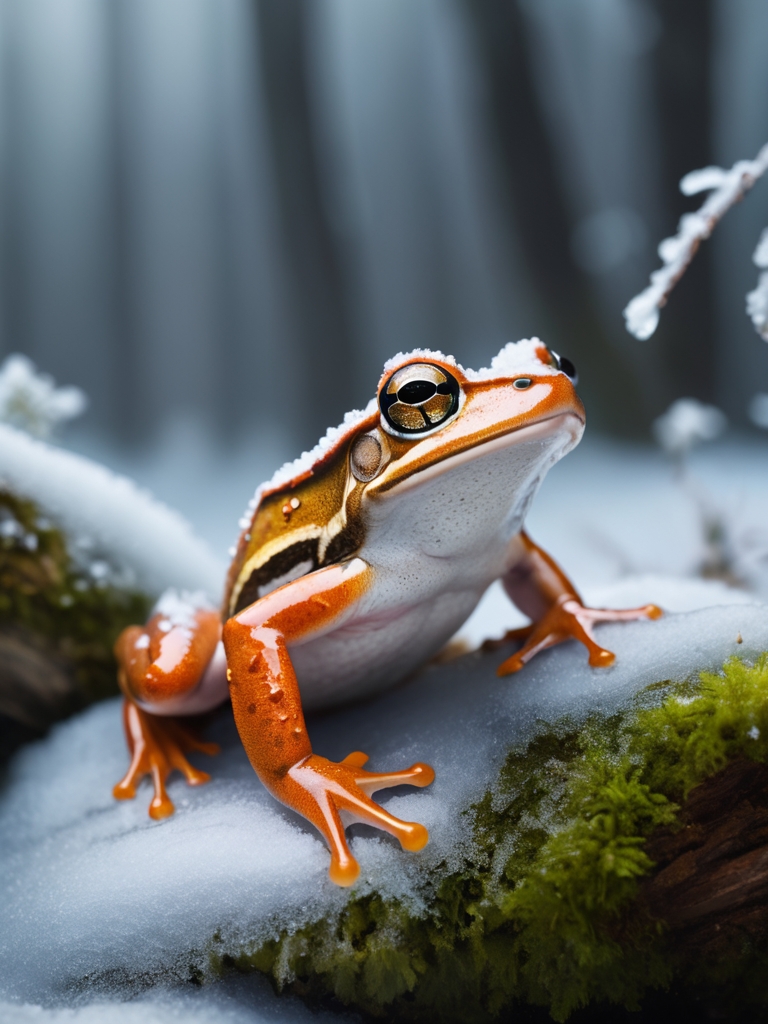
The wood frog (Rana sylvatica) exhibits a fascinating adaptation that allows it to endure harsh winter conditions. Unlike many amphibians that seek frost-free shelters, this species can survive freezing temperatures by relying on extracellular ice formation. Research has shown that while these frogs cannot tolerate extreme subzero conditions indefinitely, their natural hibernation sites—protected by leaf litter and snow—help maintain temperatures within a survivable range.
A key component of the wood frog’s survival strategy is its ability to produce glucose as a cryoprotectant. Unlike other species that accumulate glycerol or sorbitol, R. sylvatica depends almost exclusively on glucose to prevent lethal ice damage. This process is triggered by exposure to freezing temperatures, rather than gradual cooling or seasonal cues. Unlike certain insects that begin accumulating cryoprotectants well before winter, the wood frog only activates this defense when ice formation is imminent. This strategy minimizes unnecessary metabolic costs, as the conversion of stored glycogen into glucose is energetically expensive and must be reversed in spring.
The liver plays a central role in this survival mechanism. As temperatures drop, glycogen reserves in the liver rapidly break down into glucose, which is then distributed throughout the body via the bloodstream. This glucose protects cells by preventing internal ice crystallization, while extracellular water freezes in a controlled manner. Studies have shown a dramatic increase in enzyme activity within the liver during this process, particularly in those responsible for glycogen breakdown and glucose synthesis. Other tissues, including muscles and organs, exhibit relatively stable glycogen levels, suggesting that the liver alone manages cryoprotectant production.
Once frozen, the frog enters a state of suspended animation. Heartbeat, circulation, and respiration cease entirely, and the animal exists in an anoxic state, relying on stored energy reserves. During this period, metabolic activity shifts to anaerobic glycolysis, leading to the accumulation of lactic acid. While muscle tissues rely on creatine phosphate as an additional energy source, the liver directly consumes ATP to sustain a minimal energy charge. This metabolic shift helps the frog survive prolonged freezing, though its endurance is ultimately limited by the energy capacity of individual tissues.
As temperatures rise in spring, the thawing process begins. Ice melts from the inside out, circulation resumes, and metabolic activity gradually returns to normal. Remarkably, the wood frog suffers no permanent damage from this process, even after months of complete metabolic suspension. This unique adaptation makes Rana sylvatica a subject of great interest in cryobiology, particularly in fields related to organ preservation and medical applications. The frog’s ability to regulate glucose levels, despite reaching concentrations similar to those found in diabetic conditions, suggests a sophisticated hormonal control system that warrants further study.
Understanding how the wood frog survives freezing conditions provides valuable insights not only into amphibian biology but also into broader applications such as cryopreservation and medical research. As scientists continue to explore these mechanisms, Rana sylvatica remains a prime example of nature’s ability to push the boundaries of survival in extreme environments.
Conclusion
The remarkable ability of animals to adapt to winter conditions showcases the extraordinary resilience of life in the face of extreme challenges. Over millions of years, species across the globe have evolved unique physiological and behavioral mechanisms to survive harsh winter environments. From thick fur and specialized fat reserves to advanced metabolic strategies and ingenious heat conservation systems, animals have developed a range of adaptations that not only ensure survival but also allow them to thrive during periods of food scarcity, extreme cold, and limited daylight.
In cold environments like the Arctic, animals like polar bears, reindeer, and Arctic wolves exhibit highly specialized adaptations that enable them to regulate body temperature and conserve energy. Mechanisms such as counter-current heat exchange, metabolic regulation, and efficient fat utilization are key to enduring long, harsh winters without relying on constant food intake. Moreover, behavioral strategies like migration and hibernation help animals avoid the worst of the cold, ensuring they can emerge when resources are more abundant.
Aquatic animals also demonstrate remarkable thermal adaptations that enable them to survive in freezing waters, with differences in metabolic rates between Arctic and tropical species reflecting the specialized nature of life in extreme conditions. These physiological changes highlight how animals not only adjust to survive but have also mastered the art of maintaining life in environments that would otherwise be inhospitable.
The study of these adaptations offers invaluable insight into how life on Earth can endure the harshest environments. It reminds us of the intricate interconnections between life and environment, where species evolve in response to their surroundings, and how these adaptations are central to the survival and thriving of various species, regardless of the challenges they face. By understanding these incredible survival strategies, we deepen our appreciation for the complexity of nature and its unyielding capacity to adapt and endure.